Research
Introduction
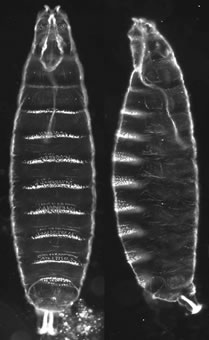
Figure 1. Mugshots of newly hatched Drosophila larvae showing the pattern elements along the anterior-posterior (AP) and dorsal-ventral (DV) axes. In both panels anterior is at top. Left panel - the ventral surface of the embryonic cuticle faces forward. Right panel - ventral is left, dorsal at right.
The long-term focus of our lab has been to understand how a single cell, the fertilized egg, develops into a multicellular organism with defined anterior-posterior and dorsal-ventral polarity and a huge variety of differentiated tissue types. In our studies we use the fruit fly, Drosophila melanogaster, which has proven to be an extremely powerful system for genetic, molecular and biochemical analysis of development. Many of the genes and pathways required for Drosophila development have been conserved throughout evolution and thus, many of the findings from Drosophila research have direct relevance to human development and health. For example, the pathway that determines dorsal-ventral polarity of the Drosophila embryo involves a cascade of serine proteases very similar to the one that regulates blood coagulation in humans. Similarly, the receptor that is required for establishing the terminal regions along the anterior-posterior axis of the embryo belongs to the receptor tyrosine kinase family, members of which are implicated in human development and disease, including cancer.
The Drosophila embryo develops very rapidly - a first instar larva (Figure 1) hatches out of the fertilized egg after only 24 hours of development. To jump start embryonic development, the mother fills the developing egg with RNA and protein molecules that are poised to act during embryogenesis. These maternally expressed RNAs and proteins provide the patterning information that tells the embryo which side is ventral and which dorsal, which end is anterior and which posterior, and where along the anterior-posterior axis its head, thorax, abdomen and tail should form. Our lab has focused primarily on dorsal-ventral axis formation but we are also interested in the maternal pathway that regulates the development of the terminal regions of the Drosophila embryo.
The Dorsal-Ventral Pathway
Figure 2. A dorsalized embryo from a female that is homozygous for a dorsal group gene mutation.
Dorsal-ventral (DV) polarity of the Drosophila embryo depends upon the activity of a group of maternally expressed genes known as the dorsal group. Females homozygous for loss-of-function mutations in any one of these genes will produce embryos that lack DV polarity and in which the fate of all cells is dorsal - these embryos exhibit a dorsalized phenotype (Figure 2). A key event in the formation of DV polarity in the Drosophila embryo is activation of the receptor encoded by the Toll gene. The Toll receptor bears similarity to several types of human receptors and although Toll is distributed uniformly in the embryonic membrane, it is activated only on the ventral side of the embryo. Ventrally restricted activation of Toll is essential for establishing the DV axis. A central and long-standing goal of our work has been to understand how spatially specific Toll activation is achieved.
The ligand for Toll is Spätzle (Spz), which is expressed in the germline and secreted into the perivitelline space surrounding the embryo as an inactive precursor. Spz is processed by the Easter protease into an active ligand for Toll only on the ventral side of the egg through the activity of a cascade of serine proteases (Figure 3).
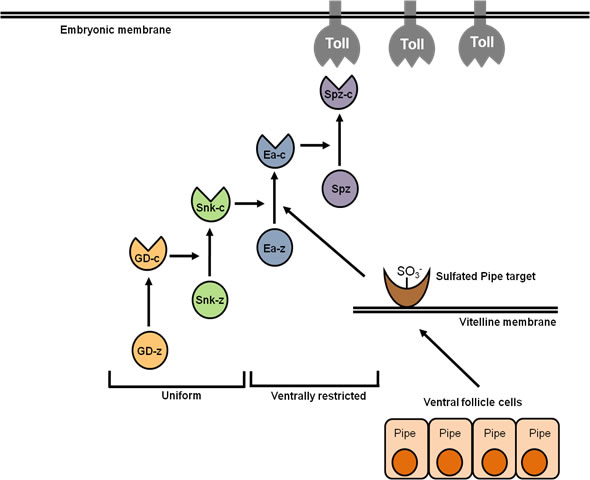
Figure 3. Serine Protease activity in the perivitelline space (between eggshell and embryo) of the Drosophila egg. Gastrulation Defective (GD) processes and activates Snake (Snk), which process and activates Easter (Ea), which processes and activates Spätzle (Spz) into the activating ligand for the Toll receptor. Processing of GD and Snk occurs uniformly throughout the perivitelline space, while processing of Ea and Spz occurs only on the ventral side of the egg. Spatially restricted processing of Ea, and therefore Spz, requires the function of the Pipe protein in the follicle cell layer that surrounds the developing egg. (z) indicates the unprocessed zymogen forms of the proteases; (c) cleaved, activated proteins. From Cho et al., 2010.
We have recently demonstrated that the protease Gastrulation Defective (GD) is localized on the ventral side of the perivitelline space (Figure 4) and that in addition to its
Figure 4. Ventral localization of GD-GFP (shown in blue) in the perivitelline space of the egg.
function in processing and activating the Snake protease, it has a second function that is required for the cleavage and activation of Easter by the Snake protease (Cho et al., 2012). Ventral localization of GD requires the activity of Pipe, a sulfotransferase that is expressed in the ventral region of the follicular epithelium that surrounds the developing egg. We have previously shown that the vitelline membrane (VM) protein Vitelline.
Membrane-Like (VML)(Figure 5) and other VM proteins are sulfated in a Pipe-dependent manner (Zhang et al., 2009). Our working hypothesis is that GD is localized to the ventral VM through an interaction with a Pipe-sulfated carbohydrate moiety present on VML and other proteins, and that this localization facilitates a productive interaction between GD, Snake and Easter that promotes ventrally specific cleavage and activation of Easter and thus of Spz. This is a very exciting finding, as it implies that the sulfation of specific carbohydrate residues provides the spatical cue that directs the formation of DV polarity in the Drosophila embryo.
Figure 5. VML localizes to the VM layer of the eggshell. A Red Fluorescent Protein-tagged version of the protein (VML-RFP) was expressed in the follicle cell layer.
The importance of carbohydrates to normal development and physiology is well known, and although the field of glycobiology is relatively new, it is rapidly moving forward due to the development of new analytic tools. The ability to combine these tools with the power of the Drosophila system to explore a specific interaction between a sulfated carbohydrate and protein function is a very promising development. A major focus of the lab is to identify these carbohydrates sulfated by Pipe and to elucidate how they influence GD function.
The study of DV axis development in Drosophila has significance to human health in a number of respects. First, this pathway represents a powerful experimental model for the study of the control of serine protease activity. Temporal and spatial regulation of serine proteases controls a vast number of medically relevant processes including blood coagulation, blood clot breakdown and complement-mediated destruction of infectious microorganisms. The Drosophila DV pathway also serves as an accessible model for the study of carbohydrate sulfation and its biological consequences. Sulfated carbohydrates affect a diverse array of medically important processes, including directed cell migration, viral, bacterial and parasitic infection and peptide growth factor signaling, yet how these sulfated molecules exert their effects remains poorly understood in most cases. The specificity of the fly DV pathway, in which a single sulfotransferase enzyme controls the induction of embryonic ventral cell fate, provides a unique experimental opportunity to define how a particular sulfate modification event exerts its biological effects. Finally, the Toll receptor was first identified through its effect on Drosophila embryo DV polarity. Drosophila Toll and the Toll-related receptors in humans play a critical role in the response to invading microorganisms via the innate immune response, a finding whose importance was recognized through the award of the 2011 Nobel Prize in Physiology or Medicine. Our proposed investigations could lead to new insights into mechanisms involved in the activation of this critically important class of receptors.
The Terminal Pathway
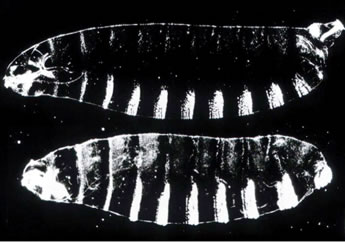
Figure 6. Top Panel - wild-type first instar larva. Bottom panel - larva from a terminal class mutant female.
The development of the terminal regions of the Drosophila embryo is dependent upon the activity of the terminal class of maternal genes. Loss-of-function mutations in these genes result in embryos that lack elements of the head and tail (Figure 6). How are the two ends defined by a single pathway? As in the DV pathway, a key gene in the terminal class pathway encodes a transmembrane receptor that is uniformly distributed in the embryonic membrane but activated in a spatially-specific manner, in this case at the ends of the embryo. This receptor, Torso, is a member of the class of receptors called receptor tyrosine kinases (RTKs), which are extremely important in human health and disease. For example, mutations in the Epidermal Growth Factor Receptor play a major role in breast cancer while mutations in the human Fibroblast Growth Factor Receptor result in more than a dozen congenital diseases affecting skeletal development.
The ligand for Torso is presumed to be encoded by the terminal class gene trunk. Like Spz in the DV pathway, Trunk is expressed in the germline and thought to be secreted as an inactive precursor into the perivitelline space that surrounds the developing embryo, where it is converted into an active ligand only at the poles. The spatial parameters of Torso activation are determined by Torsolike, which is expressed only in the polar regions of the follicular epithelium (Figure 7). Torsolike is a secreted protein that becomes localized to the polar regions of the vitelline membrane. The restriction of Torsolike expression to the poles of the follicle is essential for normal embryonic patterning; when Torsolike is ectopically expressed throughout the follicle cell layer, Torso is ectopically activated, terminal structures are expanded, and the embryos exhibit disruptions in the middle segments.
A key unanswered question is what is the role of Torsolike in Torso activation? Torsolike exhibits homology to the Membrane Attack Complex (MAC)/Perforin molecules that participate in cell killing in the immune system by embedding themselves in the cell membrane and creating pores. However, we currently do not understand how or whether this domain contributes to the function of the Torsolike protein. Ongoing efforts are directed towards understanding how Trunk activates Torso specifically at the anterior and posterior ends of the embryo and learning the role of Torsolike in this process.
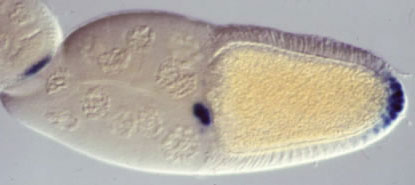
Figure 7. Expression of the torsolike gene during oogenesis in the egg chamber (follicle) in which the oocyte (future egg) develops. torsolike (blue) is expressed in two populations of follicle cells at the anterior and posterior ends of the developing egg cell.
References:
Zhang, Z., Stevens, L. M., and Stein, D. (2009). Sulfation of eggshell components by Pipe defines dorsal-ventral polarity in the Drosophila embryo. Current Biology 19, 1200-1205.
Cho, Y. S., Stevens, L. M. and Stein, D. (2010). Pipe-dependent ventral processing of Easter by Snake is the defining step in Drosophila embryo DV axis formation. Current Biology 20, 1133-1137.
Cho, Y.S., Stevens, L.M., Sievermann, K.J., Nguyen, J., and Stein, D. (2012). A ventrally localized protease in the Drosophila egg controls embryo dorsoventral polarity. Current Biology 22, 1013-1018.