Introduction
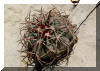
Epidermis
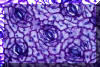
Cortex
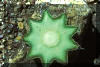
Leaves
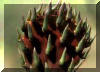
Spines
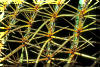
Poisons
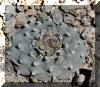
Wood
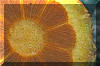
Root Succulence
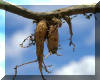
Apical Meristems
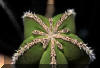
Cephalia
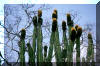
Flowers
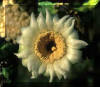
Travel
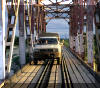
Published papers
| |
Modifications to the Cactus Cortex
The changes that
occurred in the shoot cortex during cactus evolution involved more modifications
than just adding more water-storage capacity by adding more parenchyma cells.
The evolutionary increase in width of cactus stems due to having an increasingly
wider cortex affected the selection pressures acting on numerous aspects of
cactus biology, including:
1) the need to translocate water and sugar within the
cortex itself;
2) the ability to change volume without changing surface area,
3)
the mechanical tissues needed to support the increased weight of stems, and
4)
the number of shoot apical meristem cells needed to produce the numerous cells
of the voluminous body.
Cortical bundles: The translocation
of water and sugar through the voluminous cortex
One
consequence of the increased cortex width is that the photosynthetic outer
cortex is pushed much farther away from the vascular bundles of the stem (the
cauline bundles) as the cortex differentiates. Most cacti still have a
relatively narrow pith, so in broad, globose barrel cacti and those with wide
columnar shoots, the epidermis and chlorenchyma are often several centimeters –
and as much as 30 cm in Echinocactus – from the xylem and phloem of the shoot’s vascular
bundles. Over such distances, diffusion of water is extremely slow, diffusion of
glucose or sucrose is even slower. Even with an extremely thick covering of
cuticle and wax, any epidermis will lose some water to dry desert air, and that
water must be replaced rapidly if the epidermis and outer cortex tissues are to
remain alive. Similarly, as the outer chlorophyll-bearing cortex cells produce
sugars and starch, those carbohydrates must be moved away from the plant’s
surface if they are to be of any use to the rest of the plant. Rapid
translocation throughout the voluminous cortex of many cacti is made possible by
the presence of a system of collateral cortical bundles.
Cortical bundles branch
off from stele bundles and from leaf/bud traces, then run outward, tangentially
and upward through the cortex, branching extensively and vascularizing all
regions of the cortex except the outermost layers of palisade cortex. Cortical
bundles always end near or in the basal layers of the palisade cortex, they
never extend to the hypodermis or epidermis. As with leaf venation, the most
distal portions of cortical bundles are small, narrow bundles with few
conducting cells, so that they look like leaf minor veins. In a large number of
species, cortical bundles end in clusters of cells that resemble terminal
tracheids in leaf veins: the cells are large and irregularly isodiametric but
they have helical or reticulate secondary walls reinforcing their primary walls.
These “terminal tracheid-like” cells usually occur in small clusters of about
ten to twenty cells.
Because cactus
cortex is long-lived, cortical bundles must also function for many years. In all
species, cortical bundles produce secondary phloem, and in very old bundles at
the base of very old shoots, the amount of collapsed secondary phloem can be
many millimeters thick. Many species also produce secondary xylem in their
cortical bundles, but typically it is only a small amount even in very old
bundles in very old cortex. An unusual feature that needs more study is that in
a few species, cortical bundle secondary xylem contains a matrix of xylary
fibers, not xylary parenchyma.
Because cortex
of most cacti is vascularized by cortical bundles, the epidermis and palisade
chlorenchyma can be kept hydrated and healthy no matter how much water they lose
to the dry desert air, as long as the plant itself has enough water of course.
The presence of cortical bundles may be a key innovation in the evolution of
cacti because it has permitted cactus cortex to become extremely broad as
mentioned above. It is hard to over-emphasize the importance of the evolution of
cortical bundles in cacti: whereas we animals vascularize any additional tissues
we produce (such as tumors), plants do not do this automatically. In contrast, a
plant’s ability to produce extra tissues is extremely limited by its inability
to vascularize them. Consequently other stem-photosynthetic plants such as
euphorbias, pachypodiums, stapeliads never become as broad as cacti do. It is
certainly popular to point out the convergent evolution between euphorbias and
cacti, but no euphorbia ever becomes as broad as a barrel cactus, or even as
broad as many smaller cacti. The reason is simple: none of the non-cactus
stem-photosynthetic succulents have cortical bundles in their stems. They are
free to evolve to have a wider, more voluminous cortex only up to the point at
which diffusion from the stele becomes too slow to keep the epidermis healthy.
One of the broadest euphorbias, E. obesa, achieves its width by widening
its stele: it has a broad pith that spreads its set of vascular bundles into a
ring with a wide diameter, which is in turn surrounded by a cortex that is only
moderately thick. But storing water in pith is more difficult than storing it in
the cortex because a pith can absorb a large amount of water and swell only if
the wood is flexible, otherwise either the wood would be torn apart or the pith
would be prevented from expanding enough to absorb much water.
Cortical
bundles are present in almost all members of subfamily Cactoideae (only
Blossfeldia liliputana lacks them) but are absent from subfamilies
Pereskioideae and Opuntioideae.
Mauseth, J. D. 2004. The structure of photosynthetic,
succulent stems in plants other than cacti. The International Journal of Plant
Sciences 165: 1-9.
Mauseth, J. D. 2004. Cacti and other succulents: stem anatomy
of “other succulents” has little in common with that of cacti. Bradleya 22:
131-140.
Sajeva, M., and
J. D. Mauseth. 1991. Leaflike structure in the photosynthetic, succulent stems
of cacti. Annals of Botany 68: 405 – 411.
Mauseth, J. D.,
and M. Sajeva. 1992. Cortical bundles in the persistent, photosynthetic stems of
cacti. Annals of Botany 70: 317 – 324.
Mauseth, J. D.
1993. Medullary bundles and the evolution of cacti. American Journal of Botany
80: 928 – 932.
Collapsible water-storage cells.
Cactus cortex
is divided into an inner water-storing region and an outer photosynthetic layer.
More than cortical bundles is needed to move water from the storage region to
the chlorophyllous region: water-storing cells should give up water more easily
than do other cells. The selective advantage of storing water is not just that
it keeps water-storage cells alive but rather that the water can be made
available to cells of the photosynthetic palisade cortex, the apical and
axillary buds, any flower buds or developing fruits and so on. Consequently,
water-storage cells should have thin, flexible walls that can contract or shrink
readily such that the cell’s volume diminishes as water is transferred out. On
the other hand, the cells that need the water should be more resistant to
shrinkage: if all cell walls were equally flexible, all parts of the plant would
suffer water stress equally, but that is not adaptive. Instead, water-storage
tissues should give up water so easily that the more active cells do not
experience water stress unless drought is extremely prolonged. In all cacti,
cell walls of the inner cortex are especially thin and flexible, but in many
cacti there is an additional modification: the walls are folded or undulate,
even when young and recently produced by the shoot apical meristem. Because the
walls already have folds in them, the cells are presumably set to shrink very
easily. These cells have been called collapsible cortex cells. In contrast, wall
of palisade cortex cells – the photosynthetic cells – are thicker and lack
folds, so these cannot shrink as easily. Consequently, as the volume of water
within a cactus shoot diminishes, cells of the inner cortex give up water
preferentially, transferring it to other cells, minimizing water stress and thus
allowing photosynthesis to continue.
Mauseth, J. D.
1995a. Collapsible water-storage cells in cacti. Bulletin of the Torrey
Botanical Club 122: 145 – 151.
Mucilage cells.
A perplexing
aspect of water storage in cacti and many other plants is the role of mucilage
cells. These are present in many cacti as sparsely dispersed individual cells in
some species, as such abundant cells in other species that the plants feel slimy
when cut. Spectacular concentrations of mucilage cells occur in prickly pears
(members of Opuntia with flat pads) where they form canals many
micrometers wide running just exterior to the phloem of the vascular bundles.
Plants of living stones (Ariocarpus fissuratus) also have a giant mucilage
cavity filling the center of their large taproot. Mucilage is predominately a
carbohydrate capable of binding and holding water very firmly. For example, when
samples of Opuntia are being prepared for microscopy, they are first dissected,
preserved in formaldehyde, then dehydrated by placing them is a series of
alcohol solutions, each solution being a stronger solution with less water than
the previous one (remember, beer is 5% alcohol, 95% water; wine is 14% alcohol,
86% water). When the formaldehyde is poured off so that the 25% alcohol solution
can be poured onto the samples, the mucilage has turned the formaldehyde
solution into a slimy mess that – to be really vivid – resembles a runny nose.
The mucilage is often so slimy/sticky that the tissue pieces have to be pulled
out of it with forceps, it simply will not pour off. This problem continues
until the samples have been soaked in 85% alcohol, at which point the
slimy/stickiness disappears completely. Even when the samples are exposed to 70%
alcohol (140 proof), the mucilage has such a high affinity for water that it can
hold the water in the cactus tissue, preventing its loss to the alcohol. You can
imagine that the mucilage will be just as effective in a tug-of-war with dry
desert air. Undoubtedly, mucilage does help cacti retain water.
But with water
held tightly in mucilage cells, how does that help all the other cells of the
plants? As described with collapsible cortex above, water-storage cells must
provide water to the other cells of the plant, not keep it for themselves while
the rest of the plant desiccates. One hypothesis is that as cacti experience a
prolonged drought, they should be able to digest their mucilage and thus release
the water to other cells. If so, the mucilage content of well hydrated plants
should differ from that of plants suffering from drought. However, this has not
been tested.
Mauseth, J. D.
1980. Release of whole cells of Nopalea (Cactaceae) into secretory
canals. Botanical Gazette 141: 15 – 18.
Mauseth, J. D.
1980. A stereological morphometric study of the ultrastructure of mucilage cells
in Opuntia polyacantha (Cactaceae). Botanical Gazette 141: 373 – 378.
Volume of stem must increase but
surface area of epidermis must remain constant.
The
evolutionary expansion of water-storage tissues in the cortex created problems
for the epidermis. Whereas we animals produce new epidermis cells on just about
any part of our bodies all through our lives, plants produce new epidermis cells
only at shoot and root tips or in developing leaves and flowers.
Consequently,
as a succulent stem swells to an increased volume after a rain, it can not
increase its surface area correspondingly by producing new epidermis cells. If
it were limited by the amount of stretching the epidermis could undergo without
splitting open, then the very limited ability to increase surface area would
result in a very limited absorption of water. But most cacti – and also many
other stem-succulent taxa – have a pleated surface composed of either ribs
(vertical pleats only) or tubercles (both vertical and transverse pleats).
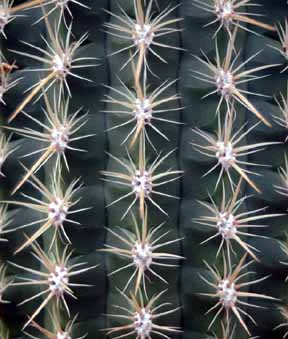 |
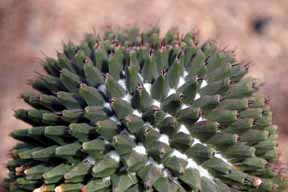 |
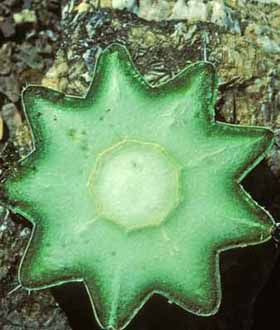 |
Three ribs on a stem of Coleocephalocereus
goebelianus. If the plant were desiccated, the bases of the ribs would
be contracted tangentially, and four or five ribs would be visible.
Click on any photo for a larger image.
|
Very short cacti like this Mammillaria
magnimamma usually have their surface folded into tubercles rather
than ribs. The tubercles allow the plant to shrink as it loses water
during a drought, or to re-expand vertically as it absorbs water after a
rain. |
Ribs are visible in this stem cross section of
this Cereus repandus. The outermost layer of cortex -- about 2 mm
thick just under the epidermis -- is dark green chlorenchyma whereas the
inner portions of the cortex are light green and mostly just store water
rather than carry out photosynthesis. |
It is easiest to think of a cactus cortex
as having two parts, an outer cortex consisting of ribs or tubercles and an
inner part between the vascular bundles and the bases of the tubercles (see the
photo above, right). After the plant has lost water during a prolonged drought,
its ribs will have less volume and the two sides of each rib will have pulled
together, thus the base of each rib will be narrow. The outer circumference of
the inner cortex is the sum of the bases of all the ribs, so with the ribs
shrunken, the outer circumference of the inner cortex is smaller, which means
that the inner cortex has become thinner through dehydration. When rains return
and the plant absorbs water, the inner cortex can swell, becoming thicker and
having a larger outer circumference. This increase in outer circumference is
accommodated by an expansion of the base of each rib: the volume of both the
inner cortex and of the ribs increases whereas the surface area of the shoot
remains unchanged. With tubercles instead of ribs, the shoots can cycle between
being shorter and taller as well as thinner and thicker. Stem-succulents with
pleated surfaces can undergo great changes in volume without any risk of their
epidermis being torn open.
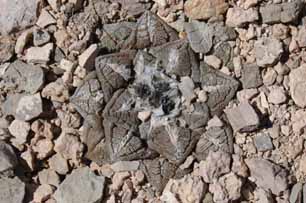 |
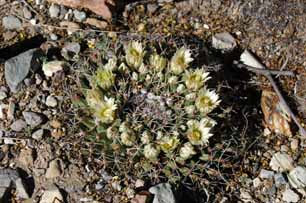 |
Plants of Ariocarpus fissuratus are never
tall, but because their surface is covered with tubercles, they can
actually contract below the soil surface if they become extremely
desiccated. Click on any photo for a larger
image. |
Many species of Mammillaria, like this
M. meiacantha, grow as short, disk-like plants. Their tubercles permit
the shoot surface to become both shorter and narrower as the plants lose
water during dry periods. |
Of course, having a pleated surface keeps the shoot from
having a minimum S/V ratio, so transpirational surface area is not as low as
possible. But the surface area is also important for light absorption, and having a pleated surface increases the
amount of light that can be absorbed as well as gives the shoot an ability to
contract and expand. As you might guess, the number and height of ribs are
important factors: many tall ribs can expand more than a few short ribs. A
curious aspect of ribs that is not immediately obvious is that whereas the total
surface area of a set of ribs is affected by both the number and height of the
ribs, the total volume of water-storage tissues located within the set of ribs
is determined only by their height, not by their number. If the number of ribs
on a shoot is doubled, then surface area is doubled but the width of the base of
each must be reduced to half, so each rib will have half the volume of an
original rib. Thus, having numerous ribs of a certain height allows more light
absorption (and water loss) than does having fewer ribs of the same height. It
might be that stem-succulents of drier habitats would have fewer ribs whereas those
of more mesic ones would have more ribs, but this still needs to be examined.
Mauseth, J. D.
2000. Theoretical aspects of surface-to-volume ratios and water-storage
capacities of succulent shoots.
American Journal of Botany 88: 1107 – 1115.
Mauseth, J. D.
& R. Ross. 1988. Systematic anatomy of the primitive cereoid cactus
Leptocereus quadricostatus. Bradleya. 6: 49 – 64.
Mauseth, J. D.
1996. Comparative anatomy of tribes Cereeae and Browningieae (Cactaceae).
Bradleya 14: 66 – 81.
Mauseth, J. D.,
and J. V. Landrum. 1997. Relictual vegetative anatomical characters in
Cactaceae: the genus Pereskia. Journal of Plant Research 110: 55 – 64.
Mauseth, J. D.,
T. Terrazas, and S. Loza-Cornejo. 1998. Anatomy of Relictual Members of
Subfamily Cactoideae, IOS Group 1a (Cactaceae). Bradleya 16: 31 – 43.
[End cortex page]
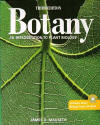
|